G-protein coupled receptors (GPCRs) are integral proteins present embedded in
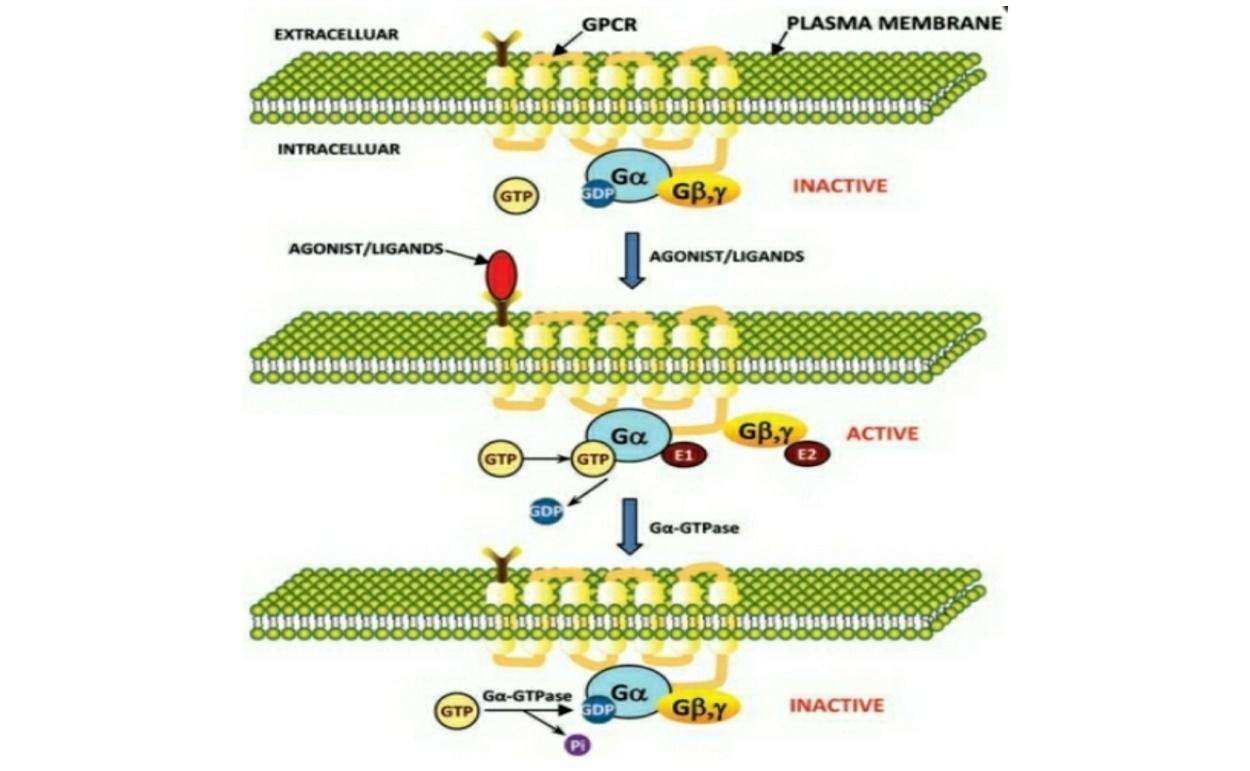
Figure 1: Model for signal transduction by activation/inactivation of heterotrimeric G-proteins through GPCR. In the inactive state heterotrimeric G-protein subunits are associated with each other. In the inactive state GDP is
Chemokine receptors belong to
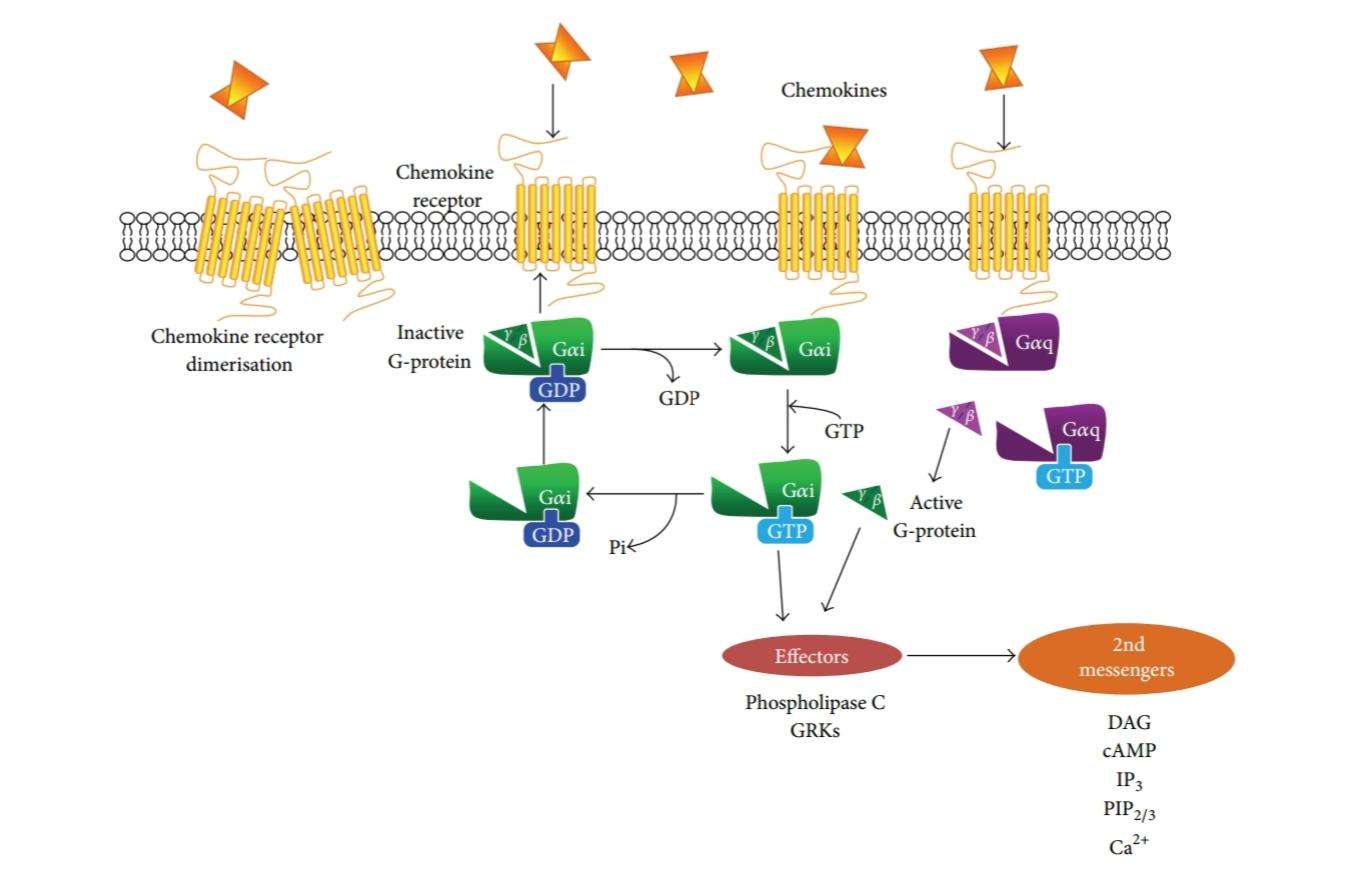
Figure 2: Schematic summary of
To date there are about 22 chemokine receptors and 50 chemokine ligands are present (Pease and Solari, 2015). Chemokine receptors are predominately expressed in the membranes of leukocytes (Figure 3) (Lodowski and Palczewski, 2009). Chemokine function plays an important role in leukocyte trafficking, cardiogenesis, neural differentiation, hematopoiesis and organogenesis (Locati, 1999). Simply, chemokine GPCR mediated coordination of leukocyte activities such as movement and positioning of leukocytes help development, maintenance and proper functioning of immune system. However, the aberrant expression of chemokine receptors and signaling contribute for assemblage of pathologies which cause improper leukocyte recruitment leading to diseases such as inflammatory, infectious, autoimmune, neurodegenerative diseases and cancers (Bennett, Fox and Signoret, 2011). Therefore, chemokine GPCRs have become an important target in therapeutic intervention and disease therapy (Doijen et al, 2017).
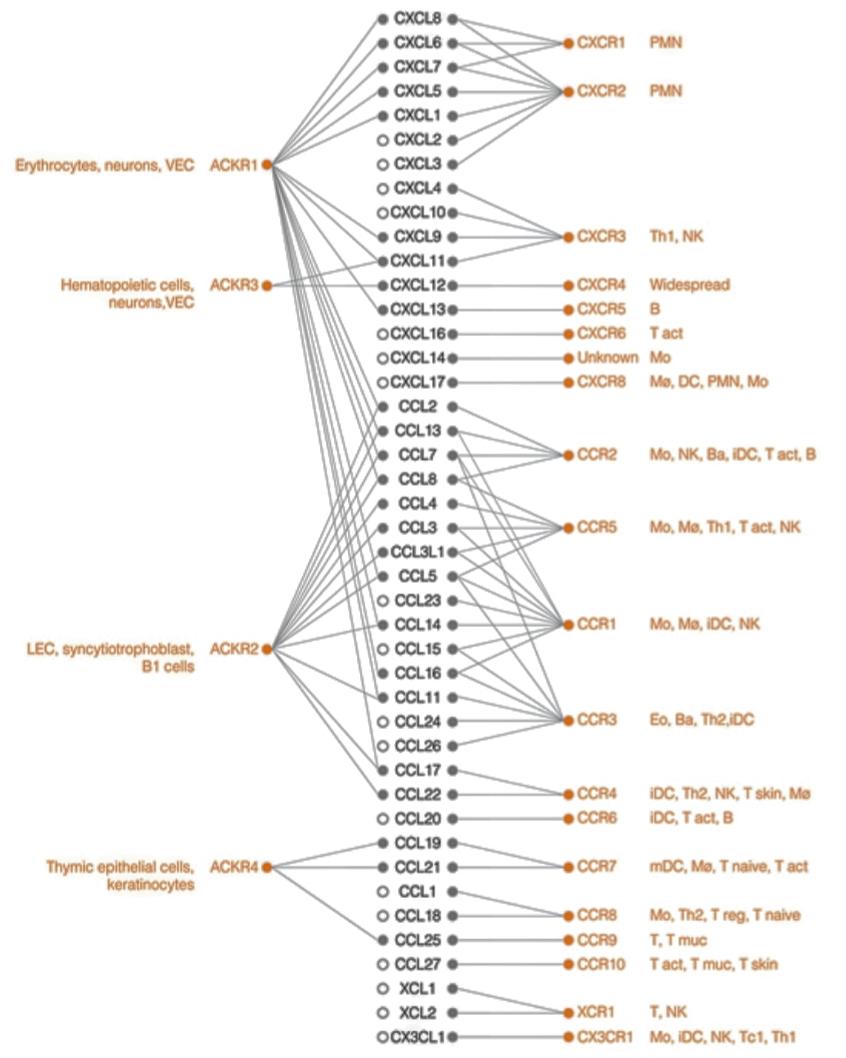
Figure 3: Leukocyte expression and specific ligand-chemokine receptor interactions (Bonecci et al, 2015).
Role of chemokine GPCRs in human immunodeficiency virus (HIV)
The chemokine GPCR, CXCR4 and CXCR3 transduce signals of their chemokine ligands CXCL12 and CXCL10 respectively. The over-expression of CXCR4 and CXCR3 have been proven to be associated with HIV progression. CXCR4 mediates HIV viral entry while CXCR3 involves in recruitment of T-cells into local HIV infected lymph nodes (Jiang, Shang and Wang, 2017; Lodowski and Palczewski, 2009; Fujii et al, 2007). T-cell trophic HIV-1 which is the etiological agent of acquired immunodeficiency syndrome (AIDS), uses CD4 and CXCR4 to assist the envelope mediated cell fusion and entry (Figure 4) (Finn and Murdoch, 2000).
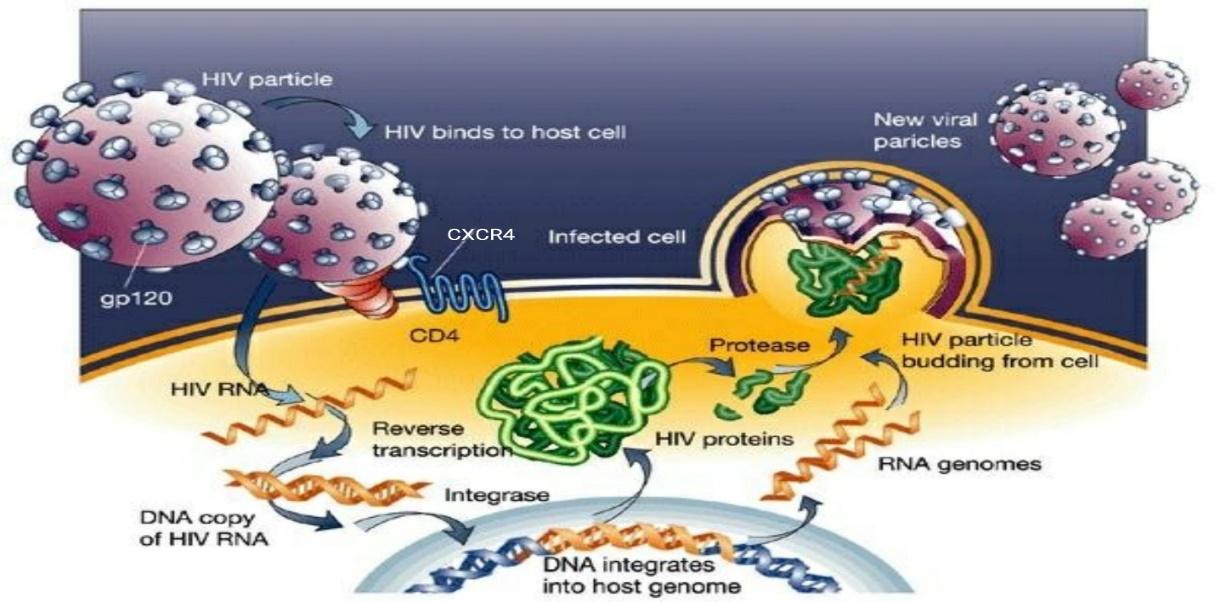
Figure 4: HIV life cycle. The entry begins with binding of the viral envelope to CD4 via envelope glycoprotein spikes present on the surface of HIV envelope. This induces conformational changes in HIV gp120 subunit exposing chemokine receptor binding site on gp120. This facilitate the binding of gp120 with CXCR4 present on the surface of the target cell. This binding of gp120 with CXCR causes further conformational changes exposing gp41 subunit. The gp41 inserts the coiled helical structures and initiate viral and target cell membrane fusion allowing the entry of viral core into the target cell. Eventually, viral RNA replicates and newly form HIV particles will be bud-off from the host cell and released into the circulation (Adapted from Jef, 2014).
Even in the absence of CD4, CXCR4 has the ability to detect the T-trophic-virus binding site. Hence CXCR4 permits HIV entry even into cells that do not express CD4. This shows that HIV uses CD4 to present the virus to the chemokine GPCR (Finn and Murdoch, 2000). The binding of natural ligand CCL12 to CXCR4 activates actin-related signaling pathway. Actin usually forms a dense network acting as a barrier to the pathogen entry. Hence, HIV gp120 competes with the natural ligand CXCL12 for CXCR4 binding and inhibits the binding of CXCL12 (Figure 5) (Jiang, Shang and Wang, 2017).
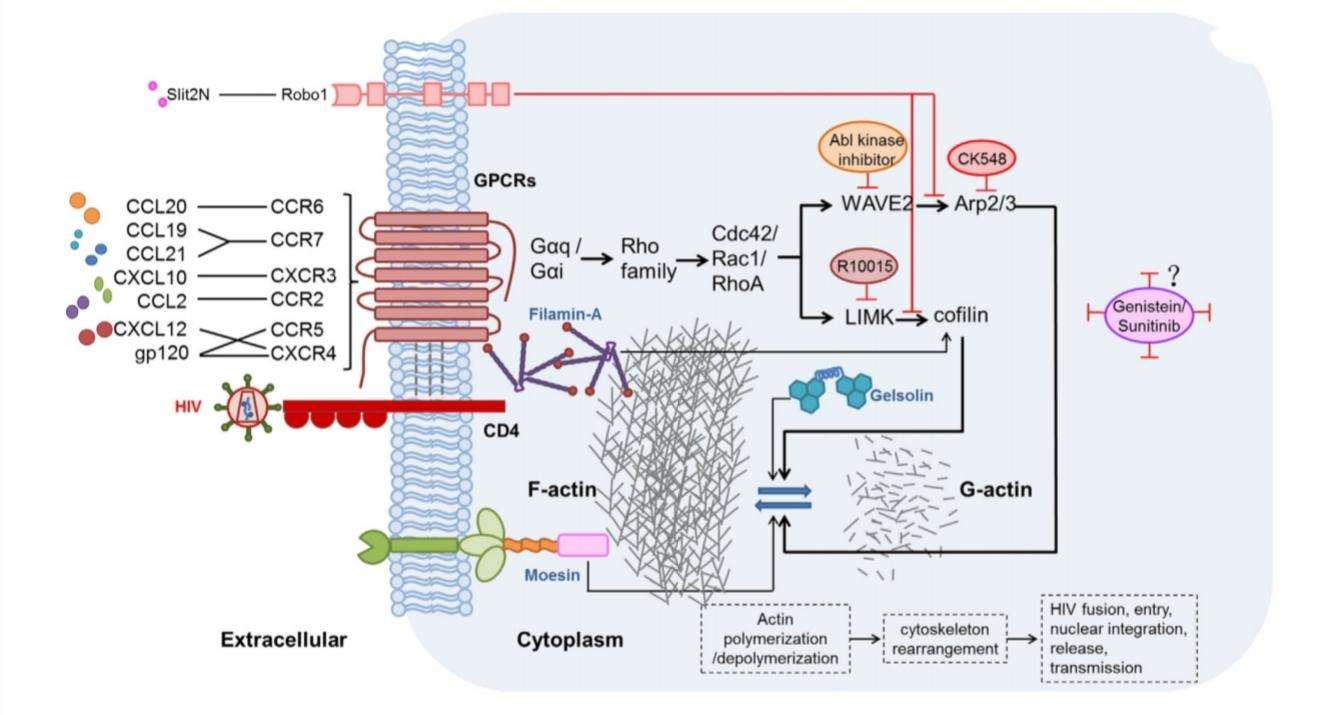
Figure 5:possible mechanisms of chemokine-induced actin activation in the promotion of HIV infection. HIV gp120 binds to CXCR4 inhibiting the interaction of CXCL12. Two major
The T-lymphocyte depletion by gp120-CXCR4 binding accelerates the HIV-1 progression. Therefore, multiple studies have been focused on therapeutic intervention by targeting the gp120-CXCR4 interaction. This approach may useful in preventing the late-stage of infection (Chan et al, 2000). To date there are several CXCR4(X4) antagonists involve in HIV-1 such as T22, T140, FC131 and KRH-3955 (Ohasi and Tamamura, 2016; Hamatake et al, 2009; Fujii et al, 1999). They competitively bind to X4 receptor preventing ligand binding to CXCR4. Thereby, inhibits the HIV-1 binding to the target cells and further progression (Figure 6). However, majority of these antagonists are at preclinical stage (Table 1) and some are suspended (AMD070, ALX40-AC) (Murakami and Yamamoto, 2010).
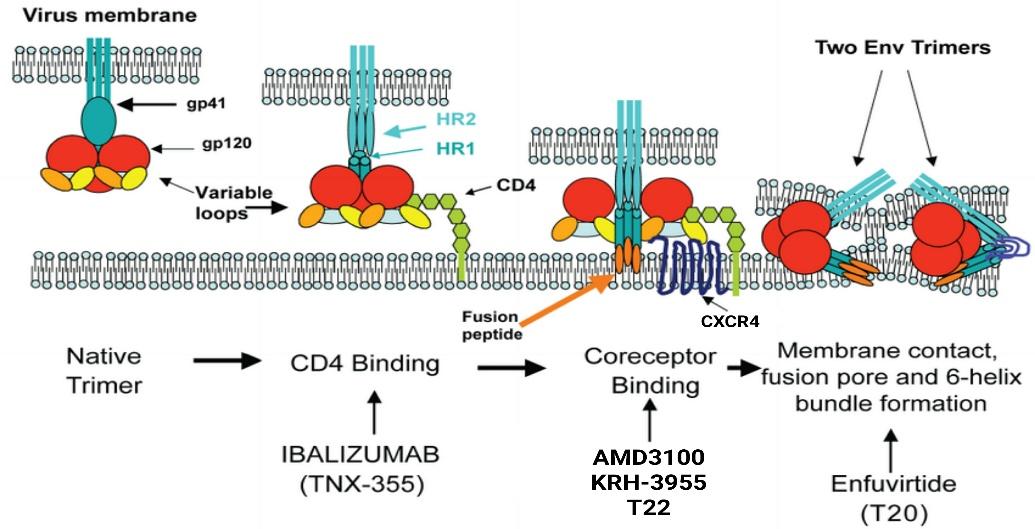
Figure 6: Therapeutic opportunities for inhibition of HIV-1 entry (Adapted from Davis et al, 2009)
Table 1: Effects of X4 HIV-1 antagonist (Adapted from Hamatake et al, 2009)
Study | Outcomes | Role | Result | Conclusion |
The novel CXCR4 antagonist KRH-3955 is an orally bioavailable and extremely potent inhibitor of HIV-1. | KRH-3955 potently and selectively inhibited the X4 HIV-1 binding and replication. | Inhibits the binding of natural ligand by competitively binding to X4 and prevents the binding of HIV-1 to CXCR4. | Figure 7: Inhibitory effects of KRH-3955 on chemokine binding to CXCR4. | KRH-3955 is a promising antagonist in X4 HIV-1 binding and an HIV-1 anti-viral agent. |
Role of Chemokine GPCRs in Glioblastoma Multiforme (GBM)
GBM is the most common and aggressive type of cancer that occurs in the brain. GBM tumors are begin in astrocytes which involve in neuronal nourishment and repair. Elevated levels of CXCR4 and CXCR7 are found to be associated with cancer stem-like cells (CSC) in GBM (Figure 8). CXCL12 is the common chemokine ligand for both CXCR4 and CXCR7 activation. The altered expression of these receptors enhance tumor survival, growth, proliferation and angiogenesis (Figure 9) (Bajetto et al, 2014; Foltz et al, 2013).
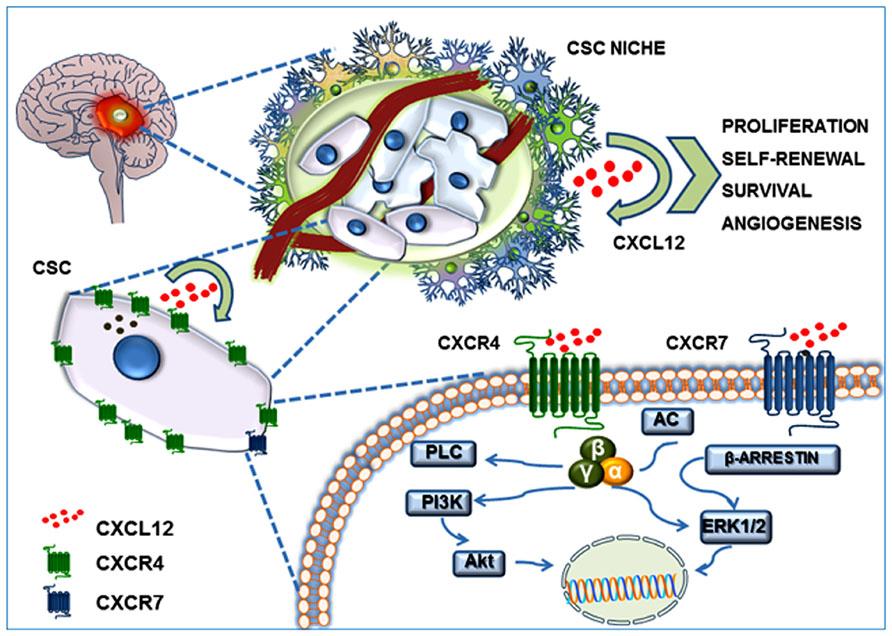
Figure 8:
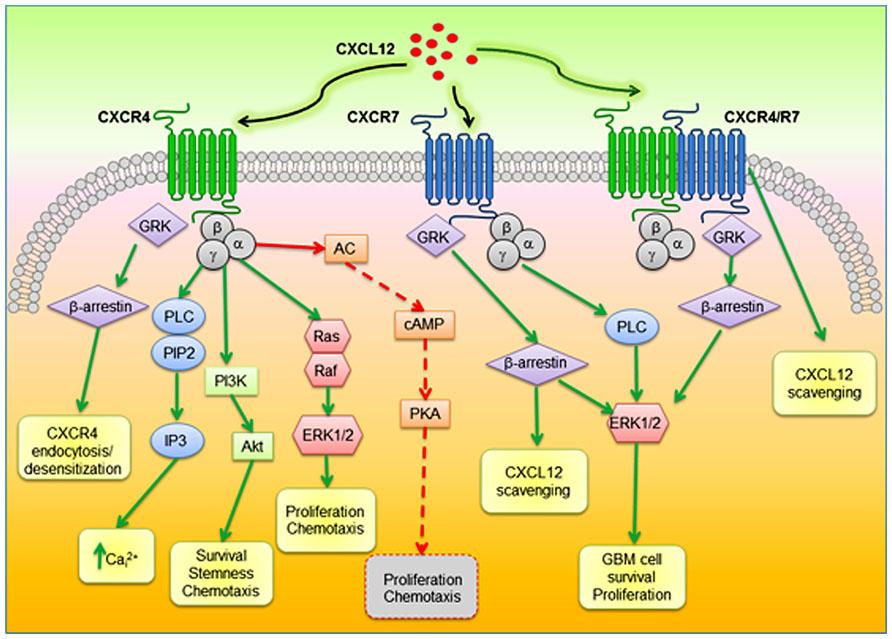
Figure 9
Table 2: CXCR4 and CXCR7 as therapeutic targets in GBM (Adapted from Bain et al, 2015; Liu et al, 2015).
Study | Outcomes | Role | Result | Conclusion |
miR-663 suppresses oncogenic function of CXCR4 in GBM | Suppressed the CXCR4 expression andmiR-663 in combination with CXCR4 antagonist (AMD3100) enhanced the tumor-suppressive effects. | Elevated oncogenic microRNA levels modulate the gene expression and increases the expression of CXCR4. miR-633 suppresses elevated microRNA levels and regulate the expression of CXCR4 while AMD3100 blocks the CXCR4 binding with ligand. | Figure 10:Upregulated miR-663 reduces the proliferation and invasion of GBM cells promoted by CXCR4 overexpression (Bain et al, 2015). | miR-633 is a potential agent in treating GBM and in combinatorial therapeutic approach. |
Targeting CXCR7 inhibits glioma cell proliferation & mobility | Suppression of CXCR7 expression by gene silencing RNA (siRNA) and CXCR7 specific antagonist CCX777 inhibits glioma cell proliferation and invasion. | Suppression of CXCR7 expression by siRNA reduces ERK ½ phosphorylation. CCX777 blocks the CXCR7. Thereby, inhibits glioma cell proliferation and invasion. | Figure 11: Knockdown of CXCR7 by siRNA (Liu et al, 2015). | Targeting CXCR7 may provide novel opportunities for improving glioma therapy. |
In summary, chemokine GPCRs diversely involve in disease progression. Therefore, they are plausible drug targets in therapeutic intervention which mainly involves in blocking the chemokine GPCRs via small molecule antagonists and monoclonal antibodies (Pease and Solari, 2015). The prototype receptor CXCR has also become an important druggable target molecule as it involves in many disease states including HIV, autoimmune, several immunodeficiency disorders and 23 types of cancers. Therefore by understanding the receptor structure, interactions and signaling pathways that involve in disease progression; safe, effective and more specific drug opportunities can be developed.
References
Bain, X., Chen, C., Cul, Y., Liu, Q., Ping, Y., Rao, J., Shi, Y., Xiao, H., Yu, S., Zhang, H. and Zhang, X. (2015) ‘miR-663 suppress oncogenic function of CXCR4 in Glioblastoma’, Clinical Cancer Research, 21(17), pp.4004-4013.
Bajetto, A., Barbieri, F., Florio, T., Harrison, J.K. and Wurth, R. (2014) ‘CXCL12 modulation of CXCR4 and CXCR7 activity in human glioblastoma stem-like cells and microenvironment’, Frontiers in Cellular Neuroscience, 8.
Bennett, L.D., Fox, J.M. and Signoret, N. (2011) ‘Mechanisms regulating chemokine receptor activity’, Immunology, 134(1), pp. 246-256.
Bonecci, R., Locati, M., Prete, A. and Sozzani, S. (2015) ‘Chemokines as effector and target molecules in vascular biology’, Cardiovascular Research, 107(3), pp. 364-372.
Chan, S.Y., Clercq, E., Connor, R.I., Goldsmith, M.A., Penn, M.L., Schols, D., Schramm, B. and Speck, RF. (2000) ‘Viral entry through CXCR4 is a pathogenic factor and therapeutic target in human immunodeficiency virus type 1 disease’, Journal of Virology, 92(7), pp. 184-192.
Chen, Y., Fang, W., Guo, D., Li, B., Liu, Y., Shang, D., Tian, L., Zhao, W. and Zhu, L. (2010) ‘Peripheral T cells derived from Alzheimer’s disease patients overexpress CXCR2 contributing to its tranendothelial migration, which is microglial TNF-α-dependent’, Neurobiology of Aging, 31(2), pp.175-188.
Davis, C., Heredia, A., Kuruppu, J.C. and Latinovic, O. (2009) ‘Pharmacotherapy of HIV-1 infection: Focus on CCR5 antagonist maraviroc’, Clinical Medicine, 1, pp.1497-1510.
Doijen, J., Haes, W. D., Landuyt, B., Loy, T.V., Luyten, W., Schols, D. and Schoofs, L. (2017) ‘Signaling propertie of the human chemokine receptors CXCR4 and CXCR7 by cellular electric impedance measurements’, PLoS ONE, 12(9), pp. 1-21.
Finn, A. and Murdoch, C. (2000) ‘Chemokine receptors and their role in inflammation and infectious diseases’, Blood, 95(10), pp.3032-3043.
Foltz, G., Hothi, P., Liu, C., Luo, D., Pham, K. and Reynolds, B.A. (2013) ‘Expression and functional heterogeneity of chemokine receptors CXCR4 and CXCR7 in primary patient derived glioblastoma’, PLoS ONE, 8(3).
Fujii, N., Hoxie, J.A., Kim, J., Koyanagi, Y., Matsumoto, A., Minoguchi, S., Murakami, T., Peiper, S.C., Shid, H., Suzuki, Y., Tamamura, H., Tanaka, Y., Waki, M., Yamamoto, N. and Zhang, T. (1999) ‘Inhibitory mechanism of the CXCR4 antagonist T22 against human immunodeficiency virus type 1 infection’, Journal of Virology, 73(9), pp.7489-7496.
Fujii, N., Masuno, H., Tamamura, H. and Tsutsumi, H. (2007) ‘Development of low molecular weight CXCR4 antagonists by exploratory structural tuning of cyclic tetra and pentapeptide scaffolds towards the treatment of HIV infection, cancer metastasis and rheumatoid arthritis’, Current Medicinal Chemistry, 14(1), pp.93-102.
Hamatake, M., Huang, W., Komano, J., Kumakura, S., Murakami, T., Okuma, K., Tanaka, R., Tanaka, Y., Toma, J., Yamamoto, N., Yamazaki, T. and Yanaka, M. (2009) ‘The novel CXCR4 antagonist KRH-3955 is an orally bioavailable and extremely potent inhibitor of human immunodeficiency virus type 1 infection: Comparative studies with AMD3100’, Antimicrobial Agents and Chemotherapy, 53(7), pp.2940-2948.
Jef, H. (2014) ‘Invitro analysis of alloreactive NK cell response against HIV patient CD4+ T-cells as a potential correlate of protection against HIV transmission’, pp. 1-66.
Jiang, Y., Shang, H. and Wang, Z. (2017) ‘Chemokines and chemokine receptors: Accomplices for human immunodeficiency virus infection and latency’, Frontiers in Immunology, 8, pp.1-12.
Liu, Y., Walter, E.C. and Walter, K.A. (2015) ‘Targeting chemokine receptor CXCR7 inhibits glioma cell proliferation and mobility’, Anticancer Research, 35(1), pp.53-64.
Locati, M. (1999) ‘Chemokines and chemokine receptors: Biology and clinical relevance in inflammation and AIDS’, Annual Review of Medicine, 50, pp.425-440.
Lodowski, D.T. and Palczewski, K. (2009) ‘Chemokine receptors and other G protein-coupled receptors’, Current Opinion in HIV and AIDS, 4(2), pp.88-95.
McNeil, E. and Patel, J. (2013) ‘The downstream regulation of chemokine receptor signaling: Implications for artherosclerosis’, Mediators of Inflammation, 5.
Murakami, T. and Yamamoto, N. (2010) ‘Role of CXCR4 in HIV infection and its potential as a therapeutic target’, Future Microbiology, 5(7), pp.1025-1039.
Ohasi, N. and Tamamura, H (2016) ‘Peptide-derived mid-sized anti-HIV agents’, Amino acids, Peptides and Proteins, 41, pp.1-29.
Pease, J.E. and Solari, R. (2015) ‘Targeting chemokine receptors in disease-a case study of CCR4’, European Journal of Pharmacology, 763, pp.169-177. PMC [Online]. DOI: 10.1016/.ehphar.2015.05.018 (Accessed: 03 March 2018).
Ransohoff, R.M. (2009) ‘Chemokines and chemokine receptors: Standing at the crossroads of immunobiology and neurobiology’, Immunity, 31(5), pp.711-721.
Tuteja, N. (2009) ‘Signaling through G protein coupled receptors’, Plant Signaling and Behavior, 4(10), pp.942–947.